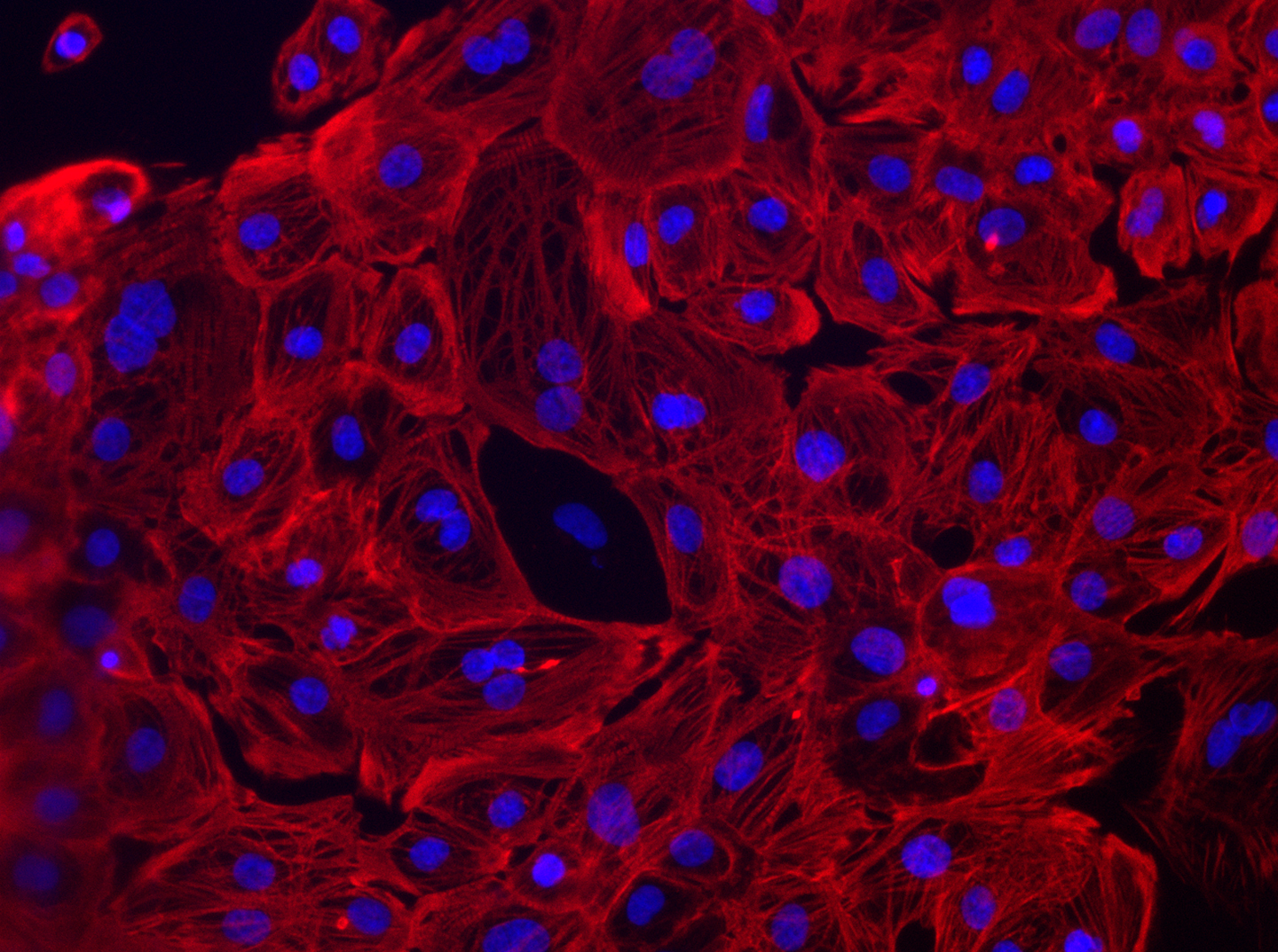
Scientific Community
Speaker Series
Additional Ventures’ virtual seminar series tackles diverse topics within and adjacent to the single ventricle field through the lens of investigators.
The Speaker Series began as a small gathering of single ventricle-centric researchers and clinicians participating in biweekly ‘Lunch & Learn’ sessions. It quickly grew into an interactive meeting of over 130 multidisciplinary investigators committed to advancing our understanding of single ventricle heart disease.
The Details
Subscribe to our mailing list to receive updates on upcoming events and opportunities.
When: Join us Wednesdays at 12 PM ET/ 9 AM PT.
Session format: A one-hour interactive Zoom meeting.
Who’s invited: This series is open to all individuals from academic, clinical, nonprofit, or government entities. Please encourage your lab members, collaborators, and colleagues to attend and participate in discussion!
How to participate: You must register to attend using your .edu, .gov, or .org email address. Registration coming soon!
TBA
We're sorry, there are no results for your query. Please try again.
Past Series
Biobanks and Biological Datasets in Single Ventricle: What’s out there?
Session Description: Shared, strong data infrastructure is critical for advancement in single ventricle science and medicine. In this session, we will discuss existing and emerging data sources for research on single ventricle heart disease, including biospecimen repositories and biological data such as sequencing, genotyping, gene expression, proteomics, metabolomics. Panelists will discuss data sharing, interoperability, and accessibility, as well as emerging possibilities for data integration and analysis.
Panelists:
Ari Cedars, MD, Johns Hopkins University
Bruce Gelb, MD, Icahn School of Medicine at Mount Sinai
Mark Russell, MD, University of Michigan
Martin Tristani-Firouzi, MD, University of Utah
Mingtao Zhao, PhD, Nationwide Children’s Hospital
Computational Fluid Dynamics: Making it a reality in everyday practice
Session Description: Surgical planning for the Fontan operation using computational fluid dynamic modeling has been around for over a decade and has contributed to our understanding of the hemodynamics of the Fontan pathway and has helped some hundred patients throughout the world. Metrics produced from this technique such as powerless and pathway resistance have been associated with exercise performance, quality of life and the development of liver fibrosis and holds the promise to intervene to improve these morbidities. Virtual surgical planning has also spawned some different configurations of the Fontan pathway such as the “Y” graft option and has cured pulmonary arteriovenous malformations. Nevertheless, computational modeling remains a “boutique” technique that is not widely used for a couple of reasons such as specialized expertise and the time needed perform it. This session will discuss what computational modeling for the Fontan is, its advantages clinically, why it has not come into widespread use and attempt to find solutions to bringing it to the masses.
Panelists:
Mark Fogel, MD, Children’s Hospital of Philadelphia
David Hoganson, MD, Boston Children’s Hospital
Alison Marsden, PhD, Stanford University
New Frontiers in Single Ventricle Gene Regulation
Session Description: Our understanding of both normal cardiac development and single ventricle molecular etiology focuses largely on regulation of gene expression at the transcriptional level. However, groundbreaking genetic, developmental, and epidemiological studies have identified key roles for RNA-based regulation of gene expression in SV models and HLHS patients. This session will (1) highlight these advances to make the case that understanding RNA-based regulatory mechanisms will lead to improved understanding of cardiac development and single ventricle genotype-phenotype relationships, (2) foster discussion among pioneers on ongoing work in understanding RNA-based regulation, and (3) introduce powerful novel resources (experimental tools, computational methods, and databases) that will empower the single ventricle research community to investigate RNA-based regulation.
Moderator:
Susan Liao, PhD, New York University
Panelists:
Muge Kuyumcu-Martinez, PhD, University of Virginia
Nathan VanDusen, PhD, Indiana University
Artificial Intelligence in Medicine: What are the challenges and opportunities?
Session Description: Artificial intelligence (AI) is poised to disrupt and revolutionize medical fields via data-driven solutions to improve patient outcome. In this session, we’ll discuss how AI-based techniques impact and improve health care for single ventricle disease patients with opportunities including disease diagnosis using AI in cardiac imaging, AI-based electrogram analysis and monitoring, benefits of AI in digital pathology in histopathological analyses, AI in early symptom prediction and identification of high-risk patients, AI in clinical workflow augmentation and hospital optimization, and AI in drug and gene treatment development. We’ll also discuss how AI tools have the potential to suffer from a host of shortcomings, including an inapplicability outside of the training domain and bias and a complex evolving relationship between clinicians and AI tools.
Moderator:
Markus Rottmann, PhD, Northwestern University
Panelists:
Collin Stultz, MD, PhD, Massachusetts General Hospital, MIT
Jagmeet Singh, MD, PhD, Massachusetts General Hospital, Harvard University
Model Organisms in Single Ventricle: What’s the Best Approach?
Session Description: Studying the mechanisms, origins, or treatments of single ventricle disease benefits from the use of model organisms. In this session, we will discuss the potential advantages and limitations of using various model organisms – including drosophila, zebrafish, chick, and murine models – to study congenital heart disease. Panelists will briefly overview how they use their model organism of choice to investigate the role of genes and function, identify opportunities and limitations of each model system, and discuss approaches to overcome species-specific constraints.
Moderator:
Luis Hernandez-Nunez, PhD, Harvard University
Panelists:
Rolf Bodmer, PhD, Sanford Burnham Prebys
Caroline Burns, PhD, Boston Children’s Hospital
Geoff Burns, PhD, Boston Children’s Hospital
Jonathan Butcher, PhD, Cornell University
Benoit Bruneau, PhD, Gladstone Institutes
Tony Firulli, PhD, Indiana University
Alison Marsden, PhD, Stanford University
Georg Vogler, PhD, Sanford Burnham Prebys
Brain Health Outcomes Among Individuals with Single Ventricle Heart Disease Across the Lifespan
Session Description: Individuals with single-ventricle physiology (SVP) now are showing significantly increased longevity with increased risks to brain health across lifespan. Factors that may contribute to neurodevelopmental and neurocognitive outcomes include: (a) genetic factors, (b) brain dysmaturation beginning in utero, (c) social and environmental factors, including early adversity, (d) acquired brain injury, and (e) medical complications related to SVP, such as arrhythmias, heart failure, and other issues which may develop over time. This session will feature two focused presentations and a moderated discussion about contemporary topics related to brain health, including: (1) associations between brain imaging findings, genetics, and neurodevelopmental and mental health outcomes; (2) neuroinformatic topics related to data-mining and harmonization to foster data sharing and leveraging existing consortia and registries; (3) social determinants of brain health among SVP patients across the lifespan and implications for research design and execution.
Presenters:
Kathie Eagleson, PhD, Children’s Hospital Los Angeles
Sarah Morton, MD, PhD, Boston Children’s Hospital
Panelists:
Kathie Eagleson, PhD, Children’s Hospital Los Angeles
Nadine Kasparian, PhD, Cincinnati Children’s Hospital
Sarah Morton, MD, PhD, Boston Children’s Hospital
Ashok Panigrahy, MD, Children’s Hospital of Pittsburgh
Caitlin Rollins, MD, Boston Children’s Hospital
Understanding and Overcoming Impostor Syndrome: Individual and Collective Strategies for Researchers
Impostor syndrome can stem from a variety of internal and systemic factors. It can affect people of all ages and genders and can be a hindrance when it comes to self-confidence and career advancement. In this training, you’ll learn specific strategies for identifying and mitigating impostor syndrome for yourself and for others. You’ll leave the training with a better understanding of why impostor syndrome occurs, how to name it when it’s present, and what you can do to overcome it in both yourself and in your workplace culture.
Building Your Network: The Relationships Every Researcher Needs to Nurture
As a researcher in academia, the importance of networking cannot be overstated: your professional network can be a powerful enabler of future career success. But forming your network takes time and intentionality: it is all about building individual relationships with a variety of people who can step up for you, provide professional guidance and feedback, and offer support, encouragement, and accountability around your career goals.
During the session, you’ll learn how you can proactively build and cultivate a network to help you achieve your professional goals. We’ll discuss questions like:
- What is your current style when it comes to professional networking?
- Which relationships are important to cultivate based on your unique career goals?
- What specific strategies can you apply to proactively cultivate advocate, mentor, collaborator, and/or colleague relationships?
- How can you overcome fear of rejection and other self-limiting beliefs that can act as barriers to networking success?
- How can you best leverage our current hybrid/virtual environment to maximize networking opportunities?
You will leave with a greater understanding of the importance of professional networking and a handful of practical strategies for how to go about doing it.
Lightning Round: Breaking Science from Students, Postdocs & Fellows
Modulation of Graft Anastomosis Orifice Opening by Graft Stiffness: A Computational Study
Ehsan Ban, PhD, Postdoctoral Researcher at Yale University (Jay Humphrey Lab)
Abstract: Blood flow is commonly redirected from the systemic to pulmonary arteries in procedures that palliate congenital cardiovascular defects, such as the Blalock–Taussig shunt procedure. Previous clinical and biomechanical studies reported the critical role of graft diameter in the balance of perfusion in the systemic and pulmonary circulations. However, the process of the formation of such anastomoses has not been the subject of biomechanics studies. Here, we developed a computational algorithm that starts from the host and graft represented by cylindrical vessels and predicts the anastomosis geometry and wall stresses resulting from the attachment of the axial cut in the host to the circular end of the shunt. We observed that anastomosis orifice opening increased by increasing shunt stiffness, following a Hill-type function. The host artery conforms to stiff synthetic grafts, whereas soft vein grafts conform to the host, accompanied by changes in attachment forces. We expect that the variation of orifice opening leads to significant hemodynamic and circulatory changes. In the future, we plan to apply this approach to specific patients and grafts and study the related clinical consequence.
Material properties are important for patch sizing in aortic arch reconstruction for single ventricle patients
Shannen Kizilski, PhD, Research Fellow at Boston Children’s Hospital (David Hoganson)
Abstract: Patch augmentation of the hypoplastic aortic arch is often a challenging part of Stage 1 single ventricle palliation. During this procedure, a biologic patch is manually shaped and sewn into the open aorta to increase its diameter to a normal size with smooth tapering to the unpatched sections. This procedure, however, is conducted when the aorta is unpressurized, so the reconstructed diameter at physiologic blood pressures is unknown until blood flow is restored after the procedure. Depending on stiffness of the patch material relative to the highly compliant aorta, the reconstructed segment might be significantly undersized or oversized compared to the surrounding vessel when pressurized. Diameter mismatch in the aorta is associated with abnormal flow and early ventricular failure. Prospective patch design to achieve the correct pressurized dimensions is achievable through computational modeling with accurate estimates of the mechanical properties of the vessel and patch. We have been developing this patch planning workflow through extensive tissue characterization and application of fundamental mechanical principles. Our growing database of aortic and biologic patch mechanical properties enables estimation of the zero-pressure configuration of patient-specific hypoplastic aortas. With this information, and with a target pressurized diameter for the reconstructed vessel, we calculate dimensions of the patch to be sewn in at the unpressurized state. Calculated patch sizes for pulmonary homograft versus pericardium are compared to dimensions obtained through the current technique of matching the unpressurized vessel diameter. Our results demonstrate the importance of considering both aortic and patch properties for proper patch sizing.
Prediction of Impending Central Line Associated Bloodstream Infections in Hospitalized Cardiac Patients: Development and Testing of a Machine-Learning Model
Siva Emani, Medical Student at Harvard Medical School and Boston Children’s Hospital (John Kheir Lab)
Abstract: To build a prediction model which identifies patients who will develop a positive central line-associated blood stream infection (CLABSI) in the next 24 hours. We collected variables potentially related to infection identification, including vital sign parameters, lab results, medication and nutrition information, prior microbiology results, and features of CVC use. Predictors were selected according to a forward stepwise approach using cross-validated area under the curve (AUC) as the inclusion metric. Logistic regression, lasso regression and random forest classifiers were built using standard machine learning techniques. We assessed model performance based on area under the curve (AUC), sensitivity, and false positive rate (FPR) of models run on an independent testing set (40%). 104,035 patient days with 399 positive cultures corresponding to 7,468 unique patients were included in the analysis. Major predictors included a prior history of infection, elevated maximum heart rate, elevated maximum temperature, elevated C reactive protein, exposure to total parenteral nutrition, and exposure to alteplase as predictors. The model identified 25% of positive cultures with an FPR of 0.11% (AUC = 0.82). A machine learning model can be used to predict 25% of patients who will develop a positive blood culture in the coming 24 hours with only 1.1/1,000 of these predictions being incorrect. Once prospectively validated, this tool may be used to diminish the incidence and duration of CLABSIs through timely removal of at-risk CVLs or earlier institution of antimicrobials.
The role of embryonic senescence in cardiac trabeculation and compaction
Audrey Ibre, PhD Student at INSERM (Michel Pucéat Lab)
Abstract: Embryonic senescence is a novel process uncovered over the last decade and found as essential for proper embryonic development. We investigated whether this cell process could occur during heart development. We detected senescent cells at different stages of mouse cardiac development. γH2AX+ and then p21+ cells were enriched in trabeculae and the number of senescent cells transiently increased from early to late stages of trabeculation/compaction. We performed single cell RNA-sequencing of high tomato+ trabeculae myocytes dissociated from E13.5 and E16.5 hearts from embryos generated by breeding SmaCreERT2 with Rosa26tdtomato mice. We identified several clusters of senescent cells in trabeculae myocytes. We uncovered news genes as well as specific cell processes and signaling pathways involved in cardiac senescence. Next, we used drugs that inhibit (Navitoclax) or exacerbate (Palbociclib) senescence to look at its impact on cardiac trabeculation and or compaction. High Resolution Episcopic Microscopy combined with Fractal analysis of embryonic hearts show that senescence has a major impact on ventricular compaction. We are using mice with cardiac conditional deletion of VHL, which feature impaired metabolic switch at mid-gestation. It will allow us to identify whether O2, mitochondrial ROS and metabolism underlie trabeculae myocyte senescence. Embryonic cardiac senescence is likely at the origin of ventricular compaction. If dysregulated, the cell process may be at the origin, of cardiac congenital diseases such as hypoplastic left ventricle and non-compaction syndrome often observed in rare metabolic diseases.
3D Bioprinted Platforms to Study Cellular and Molecular Mechanisms of Pulmonary Vein Stenosis
Martin Tomov, PhD, Research Scientist at Emory University (Vahid Serpooshan Lab)
Abstract: Pulmonary vein stenosis (PVS) is an acute pediatric cardiovascular disease that is always lethal if not treated early. While current clinical interventions (stenting and angioplasties) have shown promising results in treating PVS, they require multiple re-interventions that can lead to re-stenosis and diminished long-term efficacy. Thus, there is an unmet need to develop functional in vitro models of PVS that can enable study of its developmental triggers, provide a drug screen platform, or a phantom for clinical interventions. Patient-inspired 3D bioprinted tissue models provide a unique model to recapitulate and analyze the complex tissue microenvironment impacted by PVS and other cardiovascular pathologies. Here, we developed perfusable in vitro models of healthy and stenotic pulmonary vein by 3D reconstruction and bioprinting inspired by patient CT data. Models were seeded with human endothelial cells (ECs) to study cell state in healthy and stenotic geometries and analyzed via fluorescence microscopy and bioinformatics. Flow hemodynamics through the bioprinted vessels were quantified via Computational Fluid Dynamics (CFD) modeling and analyzed by Particle Image Velocimetry (4D-PIV). Cell growth and endothelialization were analyzed in detail and mechanical properties of the phantoms were characterized in detail. Our work here demonstrates the feasibility of bioprinting various cardiovascular cells, to create perfusable, patient inspired vascular constructs that can model in vivo geometries. Deeper understanding of vascular cell behavior in in vitro biomimetic models that incorporate tissue-like geometrical, chemical, and biomechanical ques could offer substantial insights etymology, prevention, and treatment of PVS, as well as other cardiovascular diseases.
Design of a Fetal Valve Prototype for Implantation in utero
Sanchita Bhat, MS, PhD Student at Georgia Tech, Cardiovascular Fluid Mechanics Laboratory (Lakshmi Prasad Dasi Lab)
Abstract: Single ventricle physiology (SVP) treatment consists of interventions that are palliative and not curative. Recently, prenatal intervention rates to prevent SVP have improved, but even patients who avoid SVP require or eventual valve replacement, limited by poor durability and somatic outgrowth. Thus, this project aims to develop a fully biodegradable tissue-engineered heart valve (TEHV) that will normalize the fetal hemodynamics to prevent SVP and allow the valve to grow with the patient. We manufactured a prototype using a cobalt chromium metal stent and biodegradable polycaprolactone leaflets and tested this prototype in our fetal pulse duplicator using physiological conditions using a 60/40 water/glycerin mixture. We conducted preliminary animal studies to establish the deployment system for the prototypes. Our prototype fetal valve was tested for 100 consecutive cardiac cycles and had a regurgitant fraction of 3.9% with an effective orifice area of 0.18 cm2 and a mean transvalvular pressure gradient of 3.84 mmHg. We had moderate success with the valves in animal models with minimal paravalvular leakage and mild to no stenosis as measured from echocardiography. There was trivial flow acceleration through the stent with peak velocity ~1.6 m/s. We are also experimenting with different stent designs and leaflet materials to optimize the prototype. This project is the first step to design to develop and manufacture a fully biodegradable tissue-engineered valve for use in utero. The current drawback in the lack of interventional techniques for congenital anomalies can be overcome with the progress of such a replacement device.
Next Generation: Device-based Interventions
Device for Mechanically Induced Ventricular Growth in Single Ventricle Patients
Amy Kyungwon Han, PhD, Assistant Professor in Mechanical Engineering at Seoul National University
Abstract: Current surgical palliation for single ventricle physiology involves bypassing the hypoplastic ventricle to convert the circulation into a one-pump system. Within this paradigm, most current research in myocardial biology and surgical methods is directed towards maintaining the health and function of the systemic single ventricle for as long as possible, and less attention has been paid to strategies for restoring biventricular or one-and-a-half ventricle circulation towards a true functional cure. We present a device that would induce favorable growth by exerting mechanical stimuli on the myocardial tissue of the hypoplastic ventricle to restore size and function. It is well known that mechanical forces contribute to tissue growth and remodeling in the cardiovascular system. This device-based intervention aims to promote eccentric growth to enable increased volume capacity of neonates with hypoplastic ventricles.
An in vitro study on a novel dynamic systemic-pulmonary shunt regulator
Milad Samaee, PhD, Research Engineer at the Cardiovascular Fluid Mechanics Laboratory at Georgia Tech
Abstract: The modified Blalock–Thomas–Taussig shunt (BTTS) is an aortic to pulmonary shunt, which is implanted as part of a palliative procedure for neonates with congenital heart disease to supply blood flow to the pulmonary arteries. However, a proper balance between the pulmonary and systemic flow ratio (QP:QS) has always been challenging in BTTS patients. The main goal of this study is to develop a novel device to dynamically control shunt flow by expanding a soft balloon around the shunt to restrict excessive flow to the pulmonary artery in a dynamic and programmable manner. The device will control diastolic run-off by closing the shunt during diastole. As proof of concept, the device prototype is tested on a shunt in an in vitro circulation simulator setup. The controllable linear actuator pressurizes the balloon hence the amount of fluid in the balloon dictates a certain level of resistance to the shunt. Instantaneous aortic pressure is recorded as a function of balloon resistance. Dynamic aortic flow, coronary flow, and shunt flow are measured. Results show that pinching the shunt by the device during diastole leads to drastically drop in shunt flow. As a consequence, the aortic pressure increases during diastole. Increase in aortic pressure during diastole results in having higher mean coronary perfusion up to 7%. Data shows a significant improvement in coronary perfusion by using the device. The future goal is developing a device as a sub-cutaneous implant that is wirelessly chargeable and programmable for better clinical management of patients with BTTS.
Design and development of device-based cardiac interventions
Ellen Roche, PhD, Associate Professor at the Institute for Medical Engineering and Science and the Department of Mechanical Engineering at the Massachusetts Institute of Technology
Abstract: My research is focused on device-enabled approaches to assist or augment the heart. Broadly, my group works on technologies to improve structural repair, provide active assistance and deliver biological therapy effectively. This seminar will focus on representative implantable devices that we have worked on in each of these three areas, each addressing an identified shortcoming of existing technologies. In terms of structural repair devices, I will discuss a minimally invasive delivery system for atraumatic repair of intracardiac defects and pediatric cardiac assist devices. Further, I will discuss the use of cyclically active implants to modulate immune response for use as vehicles for cell delivery and a targeted, refillable bioimplant which enables repeated local administration of biological or pharmacological delivery. Finally, I will discuss high fidelity organosynthetic testbeds and computational models designed to validate and interrogate device performance before advancing to in vivo studies.
Frontiers in Imaging & Microscopy
Quantitative 3D imaging of heart development and malformation
Sigolène Meilhac, PhD, Research Director, Unit of Heart Morphogenesis at the Institut Imagine and Institut Pasteur, Paris
Abstract: From the tubular primordium to the four-chambered organ, the heart undergoes extensive remodeling during in utero development. Genetic engineering and molecular analyses in animal models have provided insight into the gene regulatory networks of heart development. However, untangling the specific contributions of different factors requires more resolutive quantifications of the phenotype. We have developed 3D imaging procedures coupled to quantitative analyses and computer simulations of shape changes to provide novel mechanistic insight into mouse heart development. We have dissected the specific contribution of genetic factors such as Nodal, as well as of mechanical constraints in shaping the heart tube. This is relevant to structural congenital heart defects.
Morphological and Functional Characterization of Human Heart Organoids Using Optical Coherence Tomography
Chao Zhou, PhD, Associate Professor the Department of Biomedical Engineering, Washington University in St. Louis
Abstract: Organoids are self-organizing, organ-like three-dimensional (3D) cell cultures developing organ-specific cell types and functions. Organoids play an increasingly important role as in vitro models for studying organ development, disease mechanisms, and drug discovery. Recently, we developed a custom spectral-domain OCT (SD-OCT) system to study human heart organoids derived from iPSC cells. We demonstrated the capability of OCT to generate high-resolution 3D images in a fast, label-free and non-destructive manner over 30 days. The OCT imaging system and human heart organoid models are presented as novel research platforms to study heart development and disease mechanisms.
Delineating Advanced Brain Imaging Health Related Outcome in Single-Ventricle Heart Disease
Ashok Panigrahy, MD, Professor of Radiology, Bioinformatics, Bio-engineering and Developmental Biology at the University of Pittsburgh Medical Center, and Radiologist in Chief at Children’s Hospital of Pittsburgh of UPMC
Abstract: Our research harnesses the power of neuroimaging biomarkers to improve our understanding of the underpinnings of poor neurodevelopmental outcomes in patients with single ventricle disease across the lifespan. The current presentation will cover three topics with reference to on-going work in progress in the laboratory: (1) preclinical-human translational modeling (in collaboration with Dr. Cecilia Lo) in which we inter-relate high resolution episcopic microscopy of brain structures of murine cardiac single ventricle mutants to that of single ventricle heart disease patients; (2) imaging harmonization (empirical bayes) and data mining of multi-modal imaging (including non-invasive physiological imaging of cerebral blood flow); (3) improving neuroimaging access to both critically ill and diverse/low resource cohorts combing innovations in rapid/low-field MR and EEG neural biomarkers which can be deployed in the field.
Leveraging Machine Learning: From Bench to Bedside
Machine-learning dissection of congenital heart disease genetics
Katherine S. Pollard, PhD, Director of the Gladstone Institute of Data Science and Biotechnology, Investigator at the Chan Zuckerberg Biohub, and Professor in the Department of Epidemiology & Biostatistics and Bioinformatics Graduate Program at the University of California San Francisco
Abstract: Most families with congenital heart defects do not carry damaging variants in known risk genes. We will show how predictive modeling can be used to identify variants that might otherwise be overlooked. This includes structural variants that alter three-dimensional genome folding and combinations of rare protein coding variants in genes that are not individually significant. These results demonstrate that machine learning is an emerging tool for risk prediction and prioritization of loci for functional characterization to understand disease mechanisms.
Application of ML to cardiovascular medicine: unlocking the diagnostic potential of the ECG
Peter Noseworthy, MD, Cardiac Electrophysiologist and Professor of Medicine at Mayo Clinic, and Director of the Mayo Clinic Heart Rhythm and Physiologic Monitoring Laboratory
Abstract: We have spent several years developing and applying various AI algorithms that run on routine 12-lead ECGs. We have found that these algorithms can (1) scale human capabilities and (2) unlock hidden patterns in the data that expand our interpretive ability beyond human capacity.
Lightning Round: Breaking Science in Single Ventricle Disease Modeling
A computational model of cardiac growth and remodeling: Initial results and potential applications in Fontan patients
Amadeus M. Gebauer, Graduate Student at the Institute for Computational Mechanics of the Technical University of Munich
Abstract: Cardiac growth and remodeling (G&R) patterns change ventricular size, shape, and function. Biomechanical, neurohormonal, and genetic stimuli drive these patterns through changes in myocyte dimension and fibrosis. Adaptive G&R can stabilize short-term cardiac performance. Yet, by early adulthood, up to 50% of patients with a Fontan circulation are in heart failure, hypothesized to be caused by adverse G&R. We propose a novel microstructure-motivated computational model of organ- scale G&R in the heart based on the homogenized constrained mixture theory. Previous models reproduced consequences of G&R in bulk myocardial tissue by prescribing the direction and extent of growth but neglected underlying cellular mechanisms. In our model, the direction and extent of G&R emerge naturally from micromechanical turnover processes in myocardial tissue constituents and their homeostatic mechanical state. We tested our model on an idealized 3D left ventricular geometry and demonstrated that our model aims to maintain tensional homeostasis in hypertension conditions. We identified adaptive (stable) and adverse (unstable) G&R regions from varying systolic pressures and growth factors in a stability map. Further- more, we showed that our model also inherently captures the reversal of G&R after returning the systolic pressure to baseline following hypertension. The Fontan procedure dramatically improved the survival of children with single ventricle (SV) physiology, but the mechanisms of long-term heart failure remain poorly understood. Our microstructure-motivated G&R model could form the basis for a computational prediction of heart failure in SV patients. Furthermore, it could quantify the therapeutic potential of reversing remodeling, e.g., through left ventricular assist devices.
Cerebrovascular Accidents In Patients With A Left Ventricle Assist Device – The Role of Quantitative In Silico Models
Akshita Sahni, Graduate Student at the University of Colorado Boulder
Abstract: Left Ventricle Assist Devices (LVAD) have become a primary treatment choice for advanced heart failure, both as bridge-to-transplant as well as destination therapy. LVADs offer mechanical circulation support by shunting blood from failed left ventricle through a pump directly into the aorta. Despite advances in LVAD design and overall survival, they are associated with significant levels of morbidity and mortality. Stroke and cerebrovascular complications remain a leading cause of adverse outcomes post-LVAD implantation. The altered state of hemodynamics due to the LVAD pump action is intimately linked to the etiology and mechanism of stroke in LVAD patients. Yet, in-depth pre-implant understanding of stroke risks and propensity post-implant remains challenge. Here, we address this challenge through development of custom in silico models for stroke risks and mechanisms of cerebrovascular accidents, and integration of quantitative model outcomes with clinical data and patient outcomes data. We will present parametric in silico studies on the underlying mechanisms of stroke in LVAD patients, discuss results from a systematic patient cohort study for patients on LVAD support, and present perspectives on integration of such models into surgical decision making.
Shear and hydrostatic stress regulate heart valve remodeling through YAP-mediated mechanotransduction
Mingkun Wang, PhD, Postdoctoral Fellow at The Nancy E. and Peter C. Meinig School of Biomedical Engineering at Cornell University
Abstract: Congenital heart valve defects account for over 25% of all congenital heart disease, therapeutic options beyond surgical valve replacement are currently limited. Biomechanics is a driving force in valve development and disease, but how to harness it for preventing the defective development is largely unknown. Here we show that YAP, a key mechanotransduction mediator and Hippo pathway effector, responds to various mechanical forces during valvulogenesis. We first examined YAP expression during later stages of valve remodeling and found a spatiotemporal pattern correlated to the changes in mechanical environments. We then applied the shear stress on a monolayer of valvar endothelial cells (VEC) cultured in-vitro and hydrostatic stress on isolated valve explants. We found the low oscillatory shear stress and hydrostatic compressive stress promote YAP nuclear localization in VEC and valvar interstitial cells (VIC), while the high laminar shear stress and hydrostatic tensile stress restrict YAP entering nuclei in VEC and VIC. By inhibiting YAP, we altered the morphology of valve explants by limiting the VIC proliferation and enhancing the cell-cell junction of VEC. To verify the role of YAP in-vivo, we performed left and right atrial ligation in chick embryonic hearts. The surgery restricted or amplified blood flows in-vivo and induced a hypoplastic or hypertrophic valve phenotype. An inhibited YAP expression was found in the hypoplastic phenotype while a sustained YAP expression was observed in the hypertrophic phenotype. Together, we identified a mechanobiological role YAP in valve remodeling, wherein shear stress controls valve shape via YAP mediated VEC junction while the hydrostatic stress controls valve size through YAP mediated VIC proliferation.
A cardio-respiratory benchtop model which integrates respiratory biomechanics to investigate the effects of breathing on the venous flow of the Fontan circulation
Markus Horvath, Graduate Student in the Harvard-MIT Health Sciences and Technology Program
Abstract: The current preferred treatment for single ventricle physiology culminates in the Fontan circulation which connects the systemic and pulmonic vasculature in series. While it allows patients to survive with a single ventricle, the relentless hemodynamic burden triggers severe pathophysiological consequences. Despite great interest, understanding of the physiological interactions and development of support strategies remain limited which results in continuously high mid- to long term mortality rates over the past 20 years. One important example of limitation is highlighted in the current Fontan models; animal, benchtop, and computational. Recently, respiratory mechanics have been identified as a governing contributor to Fontan flow patterns and resulting reverse flow in the systemic venous return, yet currently available animal models fail to recreate the impact of respiration on the hemodynamics. Similarly, sophisticated models have been developed in vitro and in silico, but a physiologically relevant interaction of respiratory pressures is still lacking. This limits the development of therapeutic solutions. We present the development of quantitative tools that recreate the impact of respiration on the hemodynamics and serve as test platforms for interventions. In this work we introduce a physical and computational model of the Fontan physiology which mimics our natural breathing and flow mechanics to recreate the venous blood flow in the Fontan circulation. This will allow to study the effects of different breathing patterns and particular physiologies on the flow characteristics. Finally, it will serve as a platform to test different support strategies to improve the Fontan circulation.
Self-Powered Injection-Jet Fontan Circulation to Effectively Drop Caval Pressure in a Failing Fontan
Ray Prather, PhD, Senior Research Associate at the Arnold Palmer Hospital for Children
Abstract: The Fontan circulation is a fragile system in which imperfections at any one of multiple levels may compromise quality of life. Elevated inferior vena caval (IVC) pressure plays a key role in “Fontan failure”. We hypothesize that the Fontan circulation can be energized with an injection jet shunt (IJS) drawing flow directly from the aortic arch balanced by a fenestration. The IJS causes flow entrainment, leading to a clinically significant IVC pressure reduction of >3mmHg. We describe a tightly coupled multi-scale lumped parameter/computational fluids dynamics model to validate this hypothesis. A synthetic 3D-CAD model of the fenestrated total cavopulmonary connection (TCPC) was generated, with average dimensions matching those of a 2-4yo patient. The prescribed cardiac output is of about 2.3L/min with a body surface area of 0.7m2. Hemodynamics are modeled as unsteady, incompressible, turbulent, and blood is assumed non-Newtonian. Potential optimal IJS configurations were determined through a parametric sweep of several geometric design parameters such as TCPC morphology, shunt and fenestration diameter and location. A set of baseline simulations representing a failing Fontan with elevated IVC pressure (17.8mmHg) is first subjected to a fenestration enlargement to 7mm resulting in a 3mmHg IVC pressure drop but also significant reduction in systemic oxygen saturation. Addition of an IJS (2mm nozzle) to this model preserves the IVC pressure drop of 3.2mmHg and improves systemic oxygen saturation with only a small additional volume load to the ventricle (CO/Qs=1.2). Our current models demonstrate the potential salutary effect of the IJS on the Fontan circulation.
Modeling Ventricular Hypoplasia in Pulmonary Atresia with Intact Ventricular Septum Using Patient-Specific iPSCs
Yang Yu, PhD, Postdoctoral Fellow at Nationwide Children’s Hospital
Abstract: Pulmonary atresia with intact ventricular septum (PA-IVS) is a detrimental congenital heart disease where the pulmonary valve is not appropriately developed. Several hypotheses speculated to explain the pathogenesis of this disorder contains abnormal coronary arterial development, atypical blood flow through the venous valve, and atretic pulmonary valve formation. The conventional treatment strategy is pulmonary valve perforation and PA-IVS patients after treatment present with varying degrees of ventricular hypoplasia: from single ventricle palliation (1v) to 1½-ventricle palliation (1.5v) and bi-ventricle repair (2v). Mechanistic studies are required to further explain the different levels of RV hypoplasia in PA-IVS patients. Here, we generated PA-IVS-specific induced pluripotent stem cells (iPSCs) from patients with a spectrum of RV hypoplasia. PA-IVS iPSC-derived cardiomyocytes (iPSC-CMs) contracted normally and displayed sarcomeric structures with intercalated cardiac troponin T and α-actinin. Early-stage PA-IVS iPSC-CMs exhibited a variety of compromised proliferation activities, which could not be rescued by Wnt signaling pathway activation. Transcriptomic profiling by bulk RNA seq suggested that pathways involved in the cell cycle and mitosis were downregulated in day13 PA-IVS-1v iPSC-CMs, but not in PA-IVS-2v iPSC-CMs. However, at a later stage (day20), pathways involved in the regulation of cell division and mitosis were upregulated in PA-IVS-1v cardiomyocytes, indicating a possible developmental delay in the cardiomyocyte proliferation for PA-IVS-1v. Intriguingly, differentially expressed genes between PA-IVS-2v and control cardiomyocytes were primarily enriched in the pathways relevant to glucose metabolism, mitochondrial biogenesis, and muscle contraction. We conclude that patient iPSC-CMs can recapitulate cardiomyocyte proliferation defects involved in ventricular hypoplasia in PA-IVS.
Biventricular Shape Modes Discriminate Pulmonary Valve Replacement in Tetralogy of Fallot Better Than Imaging Indices
Sachin Govil, Graduate Student in the Department of Bioengineering at University of California San Diego
Abstract: Current indications for pulmonary valve replacement (PVR) in repaired tetralogy of Fallot (rTOF) rely on cardiovascular magnetic resonance (CMR) image-based indices but are inconsistently applied, lead to mixed outcomes, and remain debated. This study aimed to test the hypothesis that specific markers of biventricular shape may discriminate differences between rTOF patients who did and did not require subsequent PVR better than standard imaging indices. In this cross-sectional retrospective study, biventricular shape models were customized to CMR images from 84 rTOF patients. A statistical atlas of end-diastolic shape was constructed using principal component analysis. Multivariate regression and clustering analysis were used to test the ability of shape modes and imaging indices to discriminate PVR status as evaluated by a Matthews correlation coefficient (MCC). Geometric strain analysis was conducted to assess shape mode associations with systolic function. PVR status correlated significantly with shape modes associated with right ventricular (RV) apical dilation and left ventricular (LV) dilation (p<0.01), RV basal bulging and LV conicity (p<0.05), and pulmonary valve dilation (p<0.01). Shape modes discriminated subsequent PVR better than standard imaging indices (MCC=0.49 and MCC=0.28, respectively) and were significantly associated with RV and LV radial systolic strain. Biventricular shape modes discriminated differences between patients who did and did not require subsequent PVR better than standard imaging indices in current use. These regional features of cardiac morphology may provide insight into adaptive vs. maladaptive types of structural remodeling and point toward an improved quantitative, patient-specific assessment tool for clinical use.
Persistent Ventricle Partitioning in the Adult Zebrafish Heart
Hannah Moran, Graduate Student at the University of Colorado Anschutz Medical Campus
Abstract: The heart is the first functional organ to form in the developing vertebrate embryo. The zebrafish provides an accessible vertebrate system to study early heart morphogenesis and to gain new insights into the mechanisms of congenital disease. Although composed of only two chambers compared to the four-chambered mammalian heart, the zebrafish heart integrates the core processes and cellular lineages that are central to cardiac development across vertebrates. The vertebrate heart integrates cells from the early-differentiating first heart field (FHF) and the later-differentiating second heart field (SHF), both emerging from the lateral plate mesoderm. In mammals, this process forms the basis for the development of the left and right ventricle chambers and subsequent chamber septation. The single ventricle-forming zebrafish heart also integrates FHF and SHF lineages during embryogenesis, yet the contributions of these two myocardial lineages to the adult zebrafish heart remain incompletely understood. Here, we characterize the myocardial labeling of FHF descendants in both the developing and adult zebrafish ventricle. Expanding previous findings, late gastrulation-stage labeling using drl-driven CreERT2 recombinase with a myocardium-specific, myl7-controlled, loxP reporter results in the predominant labeling of FHF-derived outer curvature and the right side of the embryonic ventricle. Raised to adulthood, such lineage-labeled hearts retain broad areas of FHF cardiomyocytes in a region of the ventricle that is positioned at the opposite side to the atrium and encompasses the apex. Our findings are consistent with the hypothesis that integration of distinct cardiomyocyte lineages is an evolutionarily ancient trait that predates the formation of multi-chambered ventricles.
Next Generation: Modeling Single Ventricle Heart Disease
Transcriptional and morphogenetic signatures of congenital heart disease pathways
Alessandro Bertero, PhD, Associate Professor in the Department of Molecular Biotechnology and Health Sciences at the University of Turin
Abstract: Approximately half of the patients with congenital heart disease (CHD) carry potentially damaging genetic variants. Yet in most cases, a definitive association for a given mutation to disease is lacking, as is an understanding of the cell type(s) affected and the underlying mechanism. These aspects greatly limit the usefulness of genetic testing as well as the development of targeted therapies. There is, therefore, a critical need to functionally annotate the role of a growing list of candidate CHD genes. Developing and validating rapid, scalable, and predictive models of CHD is equally key. We are pursuing these goals using human pluripotent stem cell (hiPSC)-derived cardiac progenitors and their derivatives. First, we have developed a new, phenotype-agnostic pooled screening approach called OPTiKD-seq (optimized inducible knockdown deconvoluted by sequencing). This method uses single-cell RNA-sequencing (scRNA-seq) to deconvolute the global effects of barcoded loss-of-function perturbations in hPSC-derived cells. Secondly, we are studying novel self-organizing hiPSC-derived cardiac organoids (cardioids) that form endothelial cells-lined chambered models of the first or second heart field. By comparing transcriptional and morphogenic signatures induced by CHD perturbations, we aim to advance genetic testing and provide the basis for the rational development and testing of personalized CHD therapies.
Understanding Cardiac Malformations Through Targeted Cardiac Outflow Tract Mechanical Perturbation
Stephanie Lindsey, PhD, Assistant Professor in the Mechanical and Aerospace Engineering Department at the University of California San Diego
Abstract: Hemodynamics plays a vital role in early cardiac morphogenesis. Disruption of established flow patterns during critical windows of development produces a range of defects that drastically alter function of the mature heart. Malformations of the outflow tract account for over 50% of clinically relevant congenital heart defects, yet the origin of such defects remains uncertain. A major limitation in determining causality of clinically relevant cardiac abnormalities is the difficulty of studying the effects of aberrant hemodynamics alone. Here, we use a combined experimental-computational approach to study the effects of altered flow dynamics on pre-programmed great vessel morphogenesis. Through the use of targeted nonlinear optics, we nucleate and control the growth of microbubbles within outflow vessels without disturbing surrounding tissues. These targeted ablation experiments are coupled with subject-specific multiscale computational fluid dynamic models in order to track force propagation. Pressure, flow and wall shear maps obtained from these models serve as a basis for examining flow-mediated growth and adaptation in the heart and surrounding vessels. Results support a role for early great vessel hemodynamics in cardiac outflow tract rotation with wall shear stress presenting as a critical value to maintain.
Models to Understand Vascular Adaptation in Single Ventricle Heart Disease
Abhay Ramachandra, PhD, Postdoctoral Associate in Biomedical Engineering (Jay Humphrey Lab) at Yale University
Abstract: Rewiring the circulation in single ventricle palliation surgeries subject the greater thoracic vessels to an altered hemodynamic environment. Not much is known about the ensuing longitudinal vascular remodeling, including adaptation versus maladaptation. Animal models provide the advantage of isolating the effects of individual insults, probing and gaining insights into these (mal)adaptations at multiple biological scales. I will present results of vascular remodeling in response to two insults to the circulatory systems in murine models – hypoxic injury and a Glenn surgery – both relevant to single ventricle heart disease. I will elaborate on the evolution of tissue mechanics from these perturbations and some associated changes in microstructure and cell signaling. Finally, I will discuss how a multimodal data collection pipeline can inform computational models of vascular adaptation across biological scales and eventually lead to an integrative approach to guide vascular adaptations in single ventricle surgeries.
Pioneers in Modeling Cardiovascular Disease
Development of a mouse model for investigating the cardiopulmonary remodeling after the Glenn Procedure
Tai Yi, MD, Microsurgery Center Director, Nationwide Children’s Hospital
Abstract: The Glenn shunt is a surgical procedure in which the superior vena cava is anastomosed to the pulmonary artery. The Glenn procedure is the second in a series of operations resulting in the creation of the Fontan circulation in which the venous circulation is directly connected to the pulmonary artery bypassing the right ventricle. This series of staged operations represent the current standard of care for patients born with single ventricle anomalies. While life saving, the Fontan procedure is associated with decreased longevity and significant life-long morbidity. The etiology underlying the multi-organ failure associated with Fontan circulation is poorly understood but is thought to be multifactorial arising from both the genetic factors associated with the underlying single ventricle disease in addition to the pathophysiological effects of Fontan hemodynamics. Mouse models provide a powerful tool for investigating the cellular and molecular mechanisms underlying disease. Herein we describe our initial results developing a mouse model for investigating the pathophysiological mechanisms underlying the Fontan circulation beginning with cardiopulmonary remodeling after the Glenn shunt.
Modeling collateral blood flow in regenerating and failing hearts
Kristy Red-Horse, PhD, Associate Professor of Biology at Stanford University and Howard Hughes Medical Institute Investigator
Abstract: Developing organisms create tissues de novo, and the underlying instructions could inform organ regeneration. With this mindset, we study coronary arteries—which bring blood flow to heart muscle—in hopes of eventually treating coronary artery disease, the number one killer worldwide. We have discovered how mouse coronary arteries are built, and reinstated developmental pathways in adults to aid recovery following cardiac injury.
Generating the diversity of cardiovascular cell types from human pluripotent stem cells
Nicole Dubois, PhD, Associate Professor of Cell, Developmental and Regenerative Biology at the Icahn School of Medicine at Mount Sinai
Abstract: Many excellent strategies have been developed over the past years to generate the diversity of cardiovascular cell types in vitro from human pluripotent stem cells (hPSCs). However, there remain multiple open questions, including how to best mature in vitro-derived cells, how to generate complex tissue models or how to derive cells of the ventricular conduction system, for example. We show that transient Notch activation in ventricular cardiomyocytes results in stable induction of a Purkinje Fiber-like fate, including expression of conduction system markers, increased conduction velocity and adoption of Purkinje Fiber-like cell morphology. We further interrogated the role of metabolism for cardiac maturation, and have found that activation of PPAR signaling, in an isoform-specific manner, results in metabolic maturation of hPSC-derived cardiomyocytes, including enhanced fatty acid oxidation (FAO), expression of the FAO machinery, maturation of the mitochondrial network and enhanced sarcomere organization. Integrating these new strategies with existing approaches will enable the generation of relevant in vitro human models to study human heart development and disease.
Future of Single Ventricle: Computational, Cellular, and Animal Models
The Congenital Heart Disease Cardiac Atlas Project
Andrew D. McCulloch, PhD, Shu Chien Chancellor’s Endowed Chair in Engineering and Medicine at the University of California San Diego and Director of the Institute for Engineering in Medicine
Abstract: The Cardiac Atlas Project uses computational modeling and machine learning to quantify and understand the wealth of structural and functional information available in cardiac magnetic resonance (CMR) imaging exams. By building statistical atlases that characterize the variation across populations and patient cohorts we are discovering new anatomical and functional features in CMR exams from adults and children with congenital heart diseases including tricuspid atresia and hypertrophic left heart syndrome with Fontan physiology and tetralogy of Fallot. By using mechanistic multiscale computational models, we can predict how structural defects contribute to functional impairment and arrhythmia risk.
Mechanoregulation of fetal ventricular growth and maturation: emergence & complexity
Jonathan T. Butcher, PhD, Professor and Associate Director of the Nancy E. and Peter C. Meinig School of Biomedical Engineering at Cornell University
Abstract: Proper ventricular morphogenesis in humans requires the creation of bilaterally symmetric muscular pumping chambers, with trabeculated and compact domains, the latter with embedded conduction and vascular networks. Precise location and sizing of these domains is critical for gestational progression as each contributes vitally to the mechanical performance of the ensemble while sustaining further growth and maturation. While much is understood about mechanisms of early cardiogenesis, it is defects in later stages of growth and maturation that result in clinically serious malformations. While genetic manipulation has generated considerable insight into the contributions of individual cell lineages into cardiac formation, recent evidence elevates the potential that conditional signaling drives later growth and maturation events, in particular through sensation and response to their local hemodynamic environment. Our research group has developed novel experimental, imaging, and computational simulation technology to quantitatively monitor and perturb the environment of the fetal heart, using the chick animal model system. We have identified novel mechanically operated molecular switches that potentiate between growth and maturation motifs. Further, employing high resolution single cell and spatial RNA sequencing, we have further uncovered marked spatiotemporal differences in local cellular phenotype composition and signaling programs, which support local cellular composition as a previously unrecognized regulatory strategy for achieving structural heterogeneity. We here present some evidence towards this hypothesis as it relates to our efforts to understand and counteract mechanisms of ventricular malformation.
Lightning Round: Breaking Science in Cardiac Tissue Engineering & Stem Cells
Do endothelial cells promote electrical maturation of stem cell-derived cardiomyocytes?
Jessica Garbern, MD, PhD, Postdoctoral Fellow, Harvard University, Department of Stem Cell and Regenerative Biology and Pediatric Cardiologist, Boston Children’s Hospital
Abstract: Clinical translation of stem cell therapies for heart disease is limited by a risk of potentially life-threatening ventricular arrhythmias seen following cardiomyocyte delivery in large animal models. Enhancing cardiomyocyte maturation may reduce this arrhythmogenic risk by reducing automaticity of delivered cardiomyocytes. We tested whether human induced pluripotent stem cell-derived endothelial cells (iPSC-ECs) can enhance maturation and suppress automaticity of iPSC-derived cardiomyocytes (iPSC-CMs) in vitro. We found that co-culture of iPSC-ECs with iPSC-CMs significantly increased protein expression of cardiac troponin T, cardiac troponin I, Kir2.1, connexin 43, and CD36. In addition, using a stretchable mesh nanoelectronics device, we found that iPSC-ECs accelerated electrical maturation of iPSC-CMs. Using single cell RNA-seq, we identified differentially expressed surface markers that may facilitate purification of more mature iPSC-CMs. Further work will investigate whether these surface markers can identify and enrich for more mature iPSC-CM phenotypes.
Automated Vascular Design and Simulation for 3D Bioprinting
Zachary A. Sexton, Graduate Student at Stanford University Department of Bioengineering
Abstract: Incorporating perfusable vascular networks within engineered tissues and organs remains a grand challenge and precludes advancement towards artificial tissues at clinically relevant scales. Recent techniques in 3D biofabrication demonstrate the ability to manipulate living matter at resolutions and speeds necessary for viable tissues. However, there are currently no comprehensive algorithms to generate, model, and simulate biomimetic vascular networks for tissues of varying shape, vascular complexity, and perfusion conditions. Without a unified approach, efforts to vascularize tissues rely on simplified perfusion networks which poorly recapitulate pressure and flow distributions of native vasculature. We present a unified pipeline to meet this need through the open-source software package, SimVascular. This approach extends previous methods in constrained constructive optimization by partially binding local optimization routines and triaging collision repair to ensure efficient, accurate vascular generation. Further, new methods for implicit surface reconstruction are presented to efficiently vascularize nonconvex tissue and organ shapes with and without cavities. Thus, computational efficiency is maintained while perfusing more complex, biologic shapes. Resulting vascular networks can be exported for various 3D printing techniques as well as multiscale hemodynamic simulation. We demonstrate this comprehensive pipeline on a model of the ventricles to emphasize the application in whole tissue and organ vascular design planning. Additionally, model networks are presented for engineered shapes to meet desired bioreactor perfusion specifications and printing constraints.
Single Cell Multiomics Reveals Disrupted Gene Regulatory Networks in Congenital Heart Disease
Sanjeev Ranade, PhD, Postdoctoral Fellow at Gladstone Institute of Cardiovascular Disease
Abstract: A central question in developmental biology is understanding how disrupted gene regulatory networks in progenitor cells alter normal development and ultimately lead to disease. Spatiotemporal control of gene expression is essential for cell fate decisions and is coordinated in part by interactions between transcription factors (TFs) and cis regulatory elements (cREs) such as enhancers. Loss of function variants in TFs that lead to dysregulated gene expression patterns in development have been identified in patients with congenital heart defects (CHD). However, the disrupted activity of cREs within specific cardiac progenitor cells that can lead to CHD has not been systematically investigated at single cell resolution in vivo. Here, we integrated single-cell chromatin accessibility and transcriptomics to identify spatially restricted and temporally dynamic cREs in mouse heart development. We then defined dysregulated cREs in mice that lack Tbx1, a TF associated with CHD presentation in DiGeorge Syndrome. Integrated single-cell multiomics pointed to a critical role for Tbx1 in regulating cell-cell signaling pathways in second heart field progenitor cells but, surprisingly, not in differentiated OFT myocardium. Instead, we identified a specific subpopulation of neural crest cells that displayed aberrant expression of multiple members of Dlx, Fox and Etv transcription factors, which are required for patterning of outflow tract and craniofacial structures. Our work illustrates the power of single cell multiomics to uncover novel mechanistic insight into cardiac progenitor cells in CHD and paves the way for future in vitro perturbation studies targeting thousands of regulatory elements that may be involved in cardiogenesis.
Programmed Tube Bending Morphogenesis of a 3D Bioprinted Heart Tube
Jacqueline Bliley, Graduate Student at Carnegie Mellon University
Abstract: The cardiac tissue engineering field has emerged with the hope of generating whole heart organs. Contemporary approaches have focused on building the adult heart macroscopic organ structure using advanced biofabrication approaches; however, current engineered hearts display minimal contractility compared to the adult human heart. A limitation of these previous methods is the assumption that adult heart macroscopic structure will yield adult heart function. In contrast, in utero the heart develops from a linear tube that bends, loops and septates to form its three-dimensional structure and it is thought that these complex shape changes impart mechanical stresses that are critical to later heart organ structure and function. Thus, as an alternative approach, we sought to use embryonic heart morphogenesis as a guiding principle to develop whole heart organs. Here, we simulated the tube bending observed during early heart morphogenesis by inserting structural and mechanical asymmetries in 3D printed heart tubes to drive tube bending following application of cardiac fibroblast compaction forces. Heart tube bending resulted in region-specific changes in heart tube structure and function with the outer curvature of the bent tube displaying increased cardiomyocyte alignment and conduction velocity compared to the inner curvature of the bent tube. Differences in conduction paths were also observed with bent tubes initiating action potentials at the outer curvature, whereas linear tubes displayed conduction from one tube end to the other. These findings display some similarities to early heart morphogenesis and suggest that scientists should consider these morphogenetic stresses when attempting to build whole heart organs.
Umbilical vessels as potential grafts for congenital heart surgeries
Sae-Il Murtada, PhD, Associate Research Scientist at Yale University
Abstract: Infants born with a single ventricle defect undergo a series of staged surgeries over years to reconstruct the circulatory system. This allows deoxygenated blood from the veins to flow directly to the lungs without direct help from the heart. The earliest of these possible surgeries creates a shunt (called a Blalock-Taussig or BT-shunt) to route blood (from the subclavian artery) to the lungs (via a pulmonary artery) necessary for oxygenation. In order to design effective vascular grafts for use as BT-shunts, it is important to understand how mechanical properties of the graft affect vascular function over time. Here we characterized the mechanical properties of umbilical veins and arteries in mice and compared them to the subclavian and pulmonary arteries at two critical time points after birth. By using naturally occurring vessels rather than synthetic grafts, we reduce the risk of clotting and can also investigate the possibility of utilizing tissue-engineered grafts in the future. We found significant differences in mechanical properties in umbilical vessels and also regional differences between the subclavian and pulmonary arteries. Moreover, the umbilical veins and arteries displayed different contractile properties, which may have important implications when using umbilical vessels as potential grafts. We believe that these data can help improve the use of umbilical or tissue-engineered vessels as shunts in congenital heart surgeries and also lead to better mechanistic insights for human grafts in general.
Generating Artery and Vein Endothelial Cells From Pluripotent Stem Cells: A Toolkit for Stem Cell Biology and Tissue Engineering
Kevin Liu, Graduate Student at Stanford University
Abstract: The ability to generate human artery and vein endothelial cells (ECs) in vitro from human pluripotent stem cells (hPSCs) would provide a powerful platform to understand their diverse roles in health and disease and to engineer vascularized tissues. However, past efforts to convert hPSCs into ECs were lengthy (e.g., ~6-12 days of differentiation), inefficient (~10-60% of cells generated being endothelial cells), and typically generated cells that lacked clear artery or vein identity. Here we devise a strategy to generate human artery and vein ECs with high speed (within 3-4 days of hPSC differentiation) and purity (88-92% purity). Single-cell RNA-sequencing revealed stark transcriptional differences between hPSC-derived artery and vein ECs, confirming their distinct arteriovenous identities. Moreover hPSC-derived artery and vein ECs in vitro were transcriptionally similar to human fetal artery and vein ECs in vivo. Functionally, hPSC-derived artery and vein ECs could both form 3-dimensional networks in vivo and in vitro, but they differed in their functional responses to fluid flow (shear stress) and inflammation. There are thus extensive molecular and functional differences between hPSC-derived artery and vein ECs. To demonstrate the utility of these artery and vein ECs to model vascular diseases, we showed they could be infected by Biosafety-Level-4 (BSL4) viruses in vitro, which revealed new aspects of the tropism and effects of these fatal viruses. In sum, the ability to generate artery and vein ECs with unprecedented speed and efficiency will advance regenerative medicine, tissue engineering, and the modeling of vascular diseases.
Perfusion Bioreactor System for Electromechanical Stimulation of Cardiac Tissue
Jessica Herrmann, Medical Student at Stanford University
Abstract: Cardiomyocytes derived from induced pluripotent stem cells (iPSC-CMs) offer a tantalizing future of patient-specific cardiac tissue for treating congenital heart defects. Achieving the highest possible level of function of iPSC-CMs is important for deriving clinically meaningful tissues. In particular, electromechanical stimulation has been shown to enhance the contractility and maturation of iPSC-CMs in small linear microtissues. Yet, to date, no bioreactor system has been designed for electromechanically maturing larger scale and perfusable cardiac tissues. Here, we report on the design, fabrication, and testing of a perfusion bioreactor system capable of applying combined mechanical and electrical stimulation to tubular iPSC-derived cardiac tissue constructs. The bioreactor is a closed-loop system consisting of the following components: the cellular media bath, which accommodates four tissue constructs with respective graphite electrodes; five cellular media reservoirs; external tubing to connect the media reservoirs to the media bath; a pump to promote flow through the tubing; a pressure box for mechanical stimulation; and circuitry for applying the mechanical and electrical stimulation regimes. Each cylindrical construct has its own independently addressable inlet for individual luminal perfusion, while a separate network of bifurcating channels permits the external perfusion of all four conduit outer walls simultaneously under identical flow conditions. Within the bioreactor system, cardiac tissue conduits containing fibroblasts, endothelial cells, and iPSC-derived cardiomyocytes were 3D bioprinted using freeform reversible embedding of suspended hydrogels (FRESH) and cultured over a period of 24 hours. Future work will involve immunohistochemical studies and RNA sequencing to examine the effects of varying electromechanical stimulation paradigms on tissue maturation.
Understanding Mechanisms of Atrial-Ventricular Specification and Differentiation During Early Cardiac Development Through Single Cell RNA Sequencing
David Gonzalez, MD/PhD Student at Icahn School of Medicine at Mount Sinai
Abstract: The molecular mechanisms driving atrial and ventricular fate acquisition in vivo are incompletely understood. We previously identified that transient expression of Foxa2 during gastrulation specifies cardiac progenitors that give rise to ventricular but not atrial myocytes. In order to understand transcriptional mechanisms underlying early atrial and ventricular specification prior to and during the morphogenetic events leading to chamber formation, we performed single-cell sequencing (scSeq) on sub-dissected cardiac regions from Foxa2-Cre;mTmG embryos at the cardiac crescent (E8.25), primitive heart tube (E8.75) and late heart tube (E9.25) stages. We found that Foxa2 lineage-traced cells can be identified transcriptionally by expression of EGFP without the need for cell sorting, allowing for comparison of atrial/ventricular specific progenitors at early stages. Through use of RNA velocity and lineage trajectory tools we found progression towards differentiated myocardial cell types occurs primarily based on heart field progenitor identity, and that different progenitor populations contribute to ventricular or atrial identity through separate differentiation mechanisms. We further show that in utero exposure to exogenous retinoic acid, which plays a role in atrial chamber specification and acts as a teratogen during development, causes defects in ventricular chamber size. scSeq of RA-exposed embryos demonstrated dysregulation in FGF signaling in anterior second heart field cells and a shunt in differentiation towards formation of head mesenchyme, as well as defects in cell-cycle exit in myocardial committed progenitors. In summary, combining our Foxa2 lineage traced model with scSeq of healthy and RA-injected embryos provides insight into transcriptional mechanisms underlying key events during atrial/ventricular differentiation.
Next Generation in Cardiac TE & SC: Early Career Spotlight
Getting to the Heart of the Matter: Complex Genetics and Congenital Heart Disease
Casey Gifford, PhD, Assistant Professor of Pediatrics (Cardiology) at Stanford University
Abstract: The heritable component of most common diseases is thought to be multigenic in nature, arising from the complex relationships of multiple variants that are not sufficient to cause disease in isolation but contribute to pathogenesis cooperatively. Recent evidence from our lab as well as others has suggested that multigenic mechanisms may underlie congenital heart disease. Here, I’ll discuss the efforts of my lab to use human induced pluripotent stem cell differentiation to dissect the complex genetic interactions that underlie heart development and likely contribute to congenital heart disease.
Advancing the Utility and Application of Engineered Human Cardiac Tissues
Sharon Fleischer, PhD, Postdoctoral Research Scientist at Columbia University, Laboratory for Stem Cells and Tissue Engineering (Gordana Vunjak-Novakovic Laboratory)
Abstract: Engineered cardiac tissues derived from human induced pluripotent stem cells (iPSCs) are increasingly used for drug discovery, pharmacological studies, and in modeling development and disease. Here, I will discuss the milliPillar platform, a robust and versatile technology that has been developed and validated to provide a streamlined pipeline for reproduction and utilization of engineered cardiac tissues for in vitro research. In addition, I will demonstrate the utility of the platform to diagnose myocarditis and improve heart disease risk stratification for precision medicine.
Bioprinting of Perfusable Cardiac Tissues at Therapeutic Scale
Sebastien Uzel, PhD, Research Associate at Harvard University (Jennifer Lewis Laboratory)
Abstract: The ability to biomanufacture vascularized human cardiac tissues, and ultimately, full heart ventricles for repair, replacement or regeneration from patient-specific cells is a grand challenge. My talk will highlight the various methods of bioprinting, biomaterials design, and tissue assembly that our team has developed to recreate the scale, cellular density, and function of cardiac tissues from human pluripotent stem cells.
Pioneers in Cardiac Tissue Engineering & Stem Cells
Creating a Pulmonary Valve that Grows with the Child: a Story of Discovery
Robert Tranquillo, PhD, Professor of Biomedical Engineering and Chemical Engineering & Materials Science at the University of Minnesota
Abstract: We have developed a biologically-engineered tube of cell-produced collagenous matrix, which is allogeneic upon a decellularization performed prior to implantation and thus “off-the-shelf.” It is grown from donor dermal fibroblasts entrapped in a sacrificial fibrin hydrogel tube that is then decellularized using sequential detergent treatments. The resulting cell-produced matrix tube possesses physiological strength, compliance, alignment (circumferential) and growth potential, demonstrated in a growing lamb pulmonary artery replacement model because the matrix becomes a living tissue with the recipient’s cells post-implantation (Nat Comms 2016). Using the concept of a tubular heart valve, where the tube collapses inward with back-pressure between 3 equi-spaced constraints placed around the periphery to create one-way valve action, we have created a set of novel heart valves for adults and children that offer indefinite durability and growth potential, demonstrated by implantation in the growing lamb pulmonary artery for 52 weeks (Science 2021).
Evolution of Gene Regulatory Networks During Human Cardiogenesis
Eugin Destici, PhD, Assistant Project Scientist at the University of California San Diego (Neil Chi Laboratory)
Abstract: The heart, a vital organ which is first to develop, has adapted its size, structure and function in order to accommodate the circulatory demands for a broad range of animals. Although heart development is controlled by a relatively conserved network of transcriptional/chromatin regulators, how the human heart has evolved species-specific features to maintain adequate cardiac output and function remains to be defined. Here, we show through comparative epigenomic analysis the identification of enhancers and promoters that have gained activity in humans during cardiogenesis. These cis-regulatory elements are associated with genes involved in heart development and function, and may account for species-specific differences between human and mouse hearts. Supporting these findings, genetic variants that are associated with human cardiac phenotypic/disease traits, particularly those differing between human and mouse, are enriched in human-gained cis-regulatory elements. During early stages of human cardiogenesis, these cis-regulatory elements are also gained within genomic loci of transcriptional regulators, potentially expanding their role in human heart development. In particular, we discovered that gained enhancers in the locus of the early developmental regulator ZIC3 are selectively accessible within a subpopulation of mesoderm cells which exhibits cardiogenic potential, thus possibly extending the function of ZIC3 beyond its conserved left-right asymmetry role. Genetic deletion of these enhancers resulted in not only reduced early cardiac gene expression but also decreased cardiomyocyte differentiation. Overall, our results illuminate how human gained cis-regulatory elements may contribute to human-specific cardiac attributes, and provide insight into how transcriptional regulators may gain developmental roles through the evolutionary acquisition of enhancers.
State of the Science: Overview of Cardiac Tissue Engineering and Stem Cell Landscape
Stem Cells & Genomics for Precision Medicine
Joseph Wu, MD, PhD, Director of Stanford Cardiovascular Institute and Simon H. Stertzer, MD, Professor of Medicine and Radiology at Stanford University
Abstract: Recent technological advancements in multi-omics, CRISPR genome editing, and human induced pluripotent stem cells have enabled the implementation of precision medicine on an individual patient level. Here I will discuss recent advances in these technologies and how they may be used for elucidating mechanisms of cardiovascular diseases, for understanding chemotherapy-induced cardiotoxicity, and for implementing “clinical trial in a dish” concept.
Tissue Engineered Heart Valves: Where We’ve Been and Where We’re Going
John Mayer, Jr., MD, Senior Associate in Cardiac Surgery at Boston Children’s Hospital and Professor of Surgery at Harvard Medical School
Abstract: Investigations directed toward the development of a “tissue-engineered” heart valve began over 20 years ago. The initial concept was to create tissue engineered valve constructs based on biodegradable scaffolds combined with autologous cells, with the hopeful expectation that these living structures would prove to be durable and capable of growth. Early results in animals were promising, but scaling up for clinical application proved difficult. The field has evolved in several directions, and there are now initial clinical trials of acellular scaffolds as vascular conduits with the expectation that the host will repopulate these scaffolds. The history and current status of the field will be the topic of discussion.
Biology of Outcomes: Outcome Origins & Substrate-Outcome Relationship
Modeling ventricular hypoplasia in pulmonary atresia with intact ventricular septum (PA-IVS) using patient-specific induced pluripotent stem cells (iPSCs)
Mingtao Zhao, DVM, PhD and Vidu Garg, MD, Nationwide Children’s Hospital
Award Type: Innovation Fund
Abstract: Pulmonary atresia (PA) is a rare congenital heart defect (CHD) where the pulmonary valve that controls blood flow from the heart to the lungs does not properly develop. It is considered a critical CHD because surgical or catheter-based intervention is required soon after birth. In pulmonary atresia with intact ventricular septum (PA-IVS), the right ventricle (RV) does not fully develop because very little blood flows into or out of RV. PA-IVS is characterized by various degrees of RV hypoplasia and PA-IVS patients have long-term outcomes of single ventricle palliation (1v), 1 ½ ventricle palliation (1.5v), or bi-ventricular repair (2v). Mechanisms for the spectrum of RV hypoplasia in PA-IVS are unknown and difficult to fully ascribe to the absence of pulmonary valve. Currently, there are no reliable animal models to study the disease mechanisms of PA-IVS, further hindering the discovery of novel therapeutic treatments. In this study, we aim to elucidate the developmental mechanisms by which reduced biomechanical stretch suppresses cardiomyocyte proliferation and contributes to the RV hypoplasia found in PA-IVS patients. Our hypothesis is that reduced ventricular filling due to increased myocardial stiffness prevents embryonic cardiomyocyte proliferation and leads to the under development of RVs in PA-IVS patients. As human iPSC-derived cardiomyocytes (iPSC-CMs) are immature and resemble fetal-stage cardiomyocytes, we will employ PA-IVS patient-specific iPSC-CMs and engineered cyclic stretch to study how biomechanical forces alter the myocardial stiffness which further impacts early cardiomyocyte proliferation in PA-IVS.
The Genetics of Causes and Outcomes in SV CHD
Dr. Kim McBride, MD, Nationwide Children’s Hospital
Award Type: Innovation Fund
Abstract: The cause of most SV CHD is not known, nor do we understand what influences the outcome. When there is an underlying genetic diagnosis, it is frequently made after many months, sometimes when complications or additional problems have surfaced. Making a diagnosis early can affect outcome and may give insight into the prognosis. We will present how deep phenotyping and genome sequencing shortly after birth may impact care by early diagnosis.
Functional Cures: Bionic, Regenerative, & Transplantation Approaches
Piggy-back heart – a self powered Fontan assist device
Murali Padala, PhD, Emory University
Award Type: Innovation Fund
Abstract: Children surviving with a Fontan circulation lack a pump in their venous circulation, with the venous flow passively flowing into the lungs. A slightly higher pulmonary vascular resistance can impede venous flow, leading to its pooling in the vena cavae. Central venous pressure rises, and that has an adverse impact on the liver and several other organ systems. The need for mechanical devices that can assist the venous circulation in these patients is now established, but appropriate technologies are lacking that can be used in the long-term, without the risk of thrombosis and drive line infections, and do not make the child bed ridden. In this talk, I will introduce our concept of a piggy back heart, which is a self powered implantable chamber to drive Fontan circulation.
Development of a tissue engineered trans-catheter heart valve for use in the endovascular repair of single ventricle cardiac anomalies in utero
Dr Lakshmi Prasad Dasi, Georgia Tech
Award Type: Innovation Fund
Abstract: TBD
Unlocking Our Regenerative Capacity: Elucidating The Role Of LYST On Neotissue Formation In Tissue Engineered Vascular Grafts
Gabriel Mirhaidari, BS, The Abigail Wexner Research Institute at Nationwide Children’s Hospital / The Ohio State University
Award Type: Innovation Fund (Chris Breuer)
Abstract: Tissue engineered vascular grafts (TEVGs) hold great promise for advancing the care of children born with single ventricle defects by providing an autologous vessel that can grow and remodel with the patient. However, TEVG stenosis remains a challenge to clinical translation. Our bench-to-beside and back again approach has focused on utilizing small animal models to better inform the biological mechanisms behind TEVG stenosis and identify potential therapeutic interventions for use in the clinic. We serendipitously discovered that TEVGs have improved performance when implanted in Beige mice, which are defined by a mutant gene encoding lysosomal trafficking regulator (LYST) protein, and were able to reproduce this effect through anti-LYST antibody treatment. As little is known about LYST and its immunomodulation effects, we herein report a series of experiments to further elucidate the role of LYST mediated immunomodulation in TEVG neovessel development. Wild-type (C57BL6/J) mice and Beige mice are implanted with TEVGs. At 2 weeks, mice are imaged utilizing micro-PET CT to determine degree of TEVG stenosis and inflammation. TEVGs are explanted and digested for single cell RNA-sequencing with follow-up hierarchical clustering, heat map and gene ontology and pathway analyses performed. An anti-LYST locked nucleic acid (LNA) siRNA is generated to allow selective silencing of LYST and subsequently its molecular signaling pathways associated with LYST-mediated neovessel formation. An optimized dose of the LNA is administered to mice implanted with TEVGs with follow-up micro-PET CT angiography at 2-weeks to assess TEVG stenosis and inflammation. Grafts are explanted for single-cell RNA sequencing. The incidence of stenosis and inflammation, as well as results from single-cell RNA sequencing pathway analysis, is compared to results from the non LNA treated wild-type and beige mice to inform differences in molecular signaling pathways associated with LYST-mediated neotisue formation and LYST’s role in inhibiting TEVG stenosis.
Single Ventricle Clinical Sequelae: End-Organ Trajectory, Biomarkers, & Personalized Medicine
Origins and Burden of Multi-Organ Fibrosis in Patients with Single Ventricle Heart Disease
Elizabeth Goldmuntz, MD, FAAP, FACC and Jack Rychik, MD, Children’s Hospital of Philadelphia
Award Type: Innovation Fund
Abstract: Given medical and surgical advances, the majority of newborns presenting with single ventricle physiology now survive to early adulthood after staged surgical palliation to the Fontan circulation (FC). However, time has shown that the ever-growing population with single ventricle disease (SVD) develops significant complications that impact morbidity and contribute to early mortality. Excessive cardiac and multi-organ fibrosis as a systemic process is likely an important contributor. The SVD population is exposed to multiple stimuli known to promote fibrogenesis including chronic hypoxia, and in the heart, pressure and volume loads. Additional mechanisms that promote fibrogenesis in other disease states, such as dysregulation of the renin-angiotensin-aldosterone (RAA) system and serotonin receptor signaling, may also contribute in the SVD population. Given notable variability in clinical outcomes, genetic variability that modifies individual response to clinical stressors likely plays an important role. Unfortunately, the underlying mechanisms of dysregulated fibrogenesis are poorly understood and we are unable to identify those at highest risk based on genetic or biochemical profiles. Our goal is to identify genetic factors and biological mechanisms underlying this deleterious process so that the at-risk population can be identified and targeted therapeutics provided to prevent or reverse dysregulated fibrogenesis and preserve organ function. Specifically, we hypothesize that: (1) common and rare genetic variants within pathways regulating fibrogenesis contribute to individual risk, and (2) genotype and biomarker profiles will identify the SVD population at risk for mechanisms of dysregulated fibrogenesis, allowing for personalized, targeted therapeutics. To begin to identify the genetic and biological mechanisms underlying organ fibrogenesis, we will: (1) leverage a previously ascertained SVD cohort to test the association of genetic variants with myocardial fibrotic load as determined by cardiac MRI (CMR), and (2) prospectively enroll patients with SVD to better characterize multiorgan (heart, liver, kidney) fibrosis by MRI and serum biomarkers.
Shunt Audio Characteristics in Single Ventricle Infants as a Noninvasive Measure of Pulmonary Bloodflow
Saidie Rodriguez MD (other contributors: Camille Johnson, Goktug Ozmen, Omer Inan, PhD), Emory SOM and GaTech
Award Type: Innovation Fund
Abstract: Single ventricle infants with inadequate pulmonary blood flow require a systemic to pulmonary artery connection, (shunt) as initial palliation. When a patient is hypoxemic, shunt obstruction is among the differential diagnosis entertained with a high incidence of unplanned intervention. Current modalities to evaluate shunt function have limitations. There is a compelling need to innovate a noninvasive diagnostic tool to quantify the auditory characteristics of blood flow through shunts and could be done using a digital stethoscope combined with machine learning algorithms. We aim to 1) Establish audio characteristics of shunts correlating to a well-balanced circulation using various clinical parameters, and 2) Combine an acoustic signature with machine learning algorithms to improve detection of inadequate pulmonary bloodflow through the shunt.
Single Ventricle Etiology: Phenotype-Genotype Relationship & Model System Development
Ets1 in hypoplastic left heart syndrome
Shuyi Nie, Ph.D, Georgia Institute of Technology
Award Type: Innovation Fund
Abstract: Hypoplastic left heart syndrome (HLHS) is the most common cause of death in infants with congenital heart defects. Although it is clearly a genetic disease, little is known about the genetic mechanisms and pathophysiology underlying HLHS. In human, Ets1 has been identified as the genetic cause for heart defects in Jacobson Syndrome, in which HLHS is highly overrepresented. However, in mouse, loss of Ets1 leads to a series of heart defects including ventricular septal defects, non-compaction of left ventricle, and double-outlet right ventricle depending on the genetic backgrounds, but rarely HLHS. Recently, we showed that in frog, Ets1 loss leads to a heart phenotype very similar to that of human HLHS heart. The ventricular wall is thicker with a loss of ventricular trabeculation and a greatly reduced chamber size. There is also a subset of embryos with a growth arrested ventricle, suggesting that the development of HLHS-like phenotype may be a multi-step process. Using this model, we further demonstrated that Ets1 is particularly required in the cardiac mesoderm, rather than the cardiac neural crest cells for the heart development, consistent with an aortic arch artery contribution of the frog cardiac neural crest cells. Our ongoing work also indicates that the development of endocardium is impaired at Ets1 knocked down, suggesting that HLHS may result from a disrupted endocardium to myocardium signaling.
Modeling human TBX5 haploinsufficiency predicts regulatory networks for congenital heart disease:
Dr Irfan Kathiriya MD, PhD, University of California, San Francisco/Gladstone Institutes
Award Type: Innovation Fund (Benoit Bruneau)
Abstract: Haploinsufficiency of transcriptional regulators causes human congenital heart disease (CHD). However, underlying CHD gene regulatory network (GRN) imbalances are unknown. Here, we define transcriptional consequences of reduced dosage of the CHD transcription factor, TBX5, in individual cells during cardiomyocyte differentiation from human induced pluripotent stem cells (iPSCs). We discovered highly sensitive dysregulation of TBX5-dependent pathways—including lineage decisions and genes associated with heart development, cardiomyocyte function, and CHD genetics—in discrete subpopulations of cardiomyocytes. Spatial transcriptomic mapping revealed chamber-restricted expression for many TBX5- sensitive transcripts. GRN analysis indicated that cardiac network stability, including vulnerable CHD-linked nodes, is sensitive to TBX5 dosage. A GRN-predicted genetic interaction betweenTbx5 and Mef2c was validated in mouse, manifesting as ventricular septation defects. These results demonstrate exquisite and diverse sensitivity to TBX5 dosage in heterogeneous subsets of iPSC-derived cardiomyocytes, and predicts candidate GRNs for human CHDs, with implications for quantitative transcriptional regulation in disease.
Interventional Approaches
Comparative Hemodynamics of T-Junction and Y-Graft Anastomosis in the Penn State Fontan Pump
Nicolas Tobin
Pennsylvania State University, Department of Biomedical Engineering; Postdoc with Keefe Manning, PhD
Abstract: To improve outcomes in failing Fontan patients, Penn State is developing a small implanted centrifugal pump to support the heart demands and reduce pulmonary hypertension. The method of grafting the pump outlet onto the pulmonary arteries remains an important surgical consideration. Previous research has shown a Y-graft-type total cavopulmonary connection to have superior hemodynamics over a T-junction. This talk will discuss the comparative hemodynamics of a T-junction and Y-graft anastomosis of the Fontan pump outlet to patient-specific pulmonary arteries. Relevant hemodynamic parameters including dissipation of kinetic energy, wall shear stress, and pulmonary flow split will be presented.
Understanding Prenatal Brain Development in Single Ventricle to Improve Neurodevelopmental Outcome
Cynthia Ortinau, MD
Division of Newborn Medicine, Department of Pediatrics at Washington University in St. Louis School of Medicine; Assistant Professor of Pediatrics,
Abstract: Dramatic advances in the medical and surgical management of children born with single ventricle heart defects have enabled them to live longer and healthier lives. Yet, many survivors face substantial developmental delays or learning challenges that can have a major impact on long-term quality of life for children and their families. By adolescence, more than 2 out of every 3 children with single ventricle require developmental or special education services. Postnatal medical and surgical factors cannot predict which infants will face significant neurodevelopmental challenges. It is increasingly recognized that neurodevelopmental deficits result from abnormal brain development beginning during pregnancy. Our research in fetuses with congenital heart disease has shown smaller brain volumes and abnormal brain folding patterns occur by mid-gestation. Certain regions of the brain critical for long-term brain structure and function are particularly affected in fetuses with single ventricle heart disease. Abnormal prenatal brain development likely reflects several intersecting pathways. Factors such as reduced oxygen/nutrient delivery to the fetal brain from the heart defect itself, the function of the placenta, and the socioeconomic resources of the family may all impact prenatal brain development and neurodevelopment after birth. Our aim is to identify those clinical factors that improve mechanistic understanding of prenatal brain development in single ventricle and enable prediction of those at highest risk for neurodevelopmental impairments after birth. Our overarching goal is to use this information to facilitate prenatal counseling, provide targeted, early developmental services shortly after birth, and facilitate design and implementation of prenatal neuroprotective interventions.
Biomarker Discovery
Multi-Omic and Functional Metabolic Analysis Identified Dysregulated Lipid and Mitochondrial Metabolism in the Pediatric Failing Single Ventricle Heart
Anastacia (Tasha) Garcia, PhD
University of Colorado Anschutz Medical Campus, Children’s Hospital Colorado, Assistant Professor
Abstract: Congenital heart disease with single ventricle physiology (SV) encompasses a group of severe abnormalities in cardiac structure where improper development of the fetal heart results in only one functional pumping chamber. From a molecular standpoint, it is not well understood how the SV myocardium adapts to the chronic altered hemodynamic conditions of SV physiology, and cardiac dysfunction and ultimately heart failure (HF) are a common complication in the SV population. The purpose of this study was to characterize the transcriptomic, metabolomic, and lipidomic profiles in SV myocardium from both failing (SVHF) and non-failing (SVNF) SV patients compared to biventricular NF controls (BVNF). Furthermore, we conducted high-resolution respirometry (Oroboros Oxygraph system) to assess myocardial mitochondrial respiratory function in each of these populations. Lastly, we measured carnitine palmitoyltransferase (CPT) activity, a mitochondrial enzyme that allows the uptake of long-chain fatty acids for their subsequent oxidation. Multi-omics pathway analysis demonstrated multiple pathways that are similarly dysregulated in SVNF and SVHF, while pathways involved in mitochondrial and lipid metabolism were significantly dysregulated specifically in the SVHF population. Moreover, functional mitochondrial oxygen flux and CPT activity were significantly decreased in SVHF relative to BVNF controls. Therefore, these results provide new insights into SVHF by identifying unique gene, metabolite and lipid changes, including those related to mitochondrial metabolic function, which may serve as potential therapeutic targets for the treatment or prevention of HF in the SV population. These data are corroborated by the significant decrease seen in functional assessments of mitochondrial oxygen flux and CPT activity. Based on these findings, we have also recently begun to explore circulating peripheral mononuclear cells a potential biomarkers of myocardial mitochondrial function in the SV population. We propose that peripheral blood mononuclear cells (PBMCs) isolated from children with SV could serve as surrogate circulating molecular biomarkers for myocardial mitochondrial alterations and provide predictive prognostic value to this unique patient population. Our preliminary data demonstrates PBMCs from SVHF patients display significant changes in mitochondrial oxygen flux manifesting in decreased respiratory capacity, ATP production, and coupling efficiency, and increased reactive oxygen species relative to controls. Therefore, we hypothesize that myocardial mitochondrial metabolism represents a biomarker of disease progression in SV patients, and that patient-derived PBMCs serve as a proxy for myocardial cellular respiration.
Predicting Outcomes in Single Ventricle Heart Disease Through Circulating miRNAs
Stephanie Nakano, MD
Assistant Professor, Pediatric Cardiology; Children’s Hospital Colorado
Abstract: Single ventricle congenital heart disease (SV) is universally fatal without intervention and is the leading cause of cardiovascular death in infancy. Currently, the most common management strategy for SV is a series of palliative surgeries. While these surgeries represent significant advances in medical care, mortality remains high, with 30% of patients dying in the first year of life. Importantly, the ability to predict which infants will do well with surgical palliation is lacking.
Circulating miRNAs are increasingly recognized as effective biomarkers in a broad range of medical disciplines, aiding in both diagnosis and prognosis. Our hypothesis was that circulating miRNA profiles prior to surgical palliation may correlate with SV survival at one year and assist with risk-stratification in this population. Serum samples from subjects with SV (of right ventricular morphology) were obtained at the following time points: Pre-Norwood (n=71), Pre-Glenn (n=46) and Pre-Fontan (n=25). Outcomes were classified as alive versus death or heart transplant listing by one year of age. Serum was subject to three freeze/heat cycles to maximize miRNA release, then miRNAs were reverse transcribed using a pool of primers specific for each miRNA. Real-time PCR was performed in 384-well plates containing sequence-specific primers and TaqMan probes in the ABI7900HT. Analysis of Pre-Norwood samples demonstrated downregulation of miR-15b, -192, and 193b in patients who died or required heart transplant listing (n=22) compared to those who were alive (n=49) at one year of age. Additionally, miR-let-7b, -26a, and -454 were differentially expressed between the Pre-Norwood, Pre-Glenn, and Pre-Fontan groups, suggesting an association with surgical stage and/or age. Circulating miRNA profiles are distinct in pediatric SV patients at each surgical stage, and demonstrate promise as prognostic biomarkers of 1-year outcome in the Pre-Norwood SV population.
Tissue Engineering Approaches & Applications
Engineering In Vitro Vascularized Tissues Using FRESH 3D Bioprinted Collagen Scaffolds
Andrew Hudson, MS
Carnegie Mellon University, Regenerative Bioaterials Therapeutic Group (Adam Feinberg PhD), PhD Student
Abstract: One of the foremost challenges remaining to engineer large (>1 cm3), transplantable tissues is creating the microvasculature necessary to promote cell viability and eventual tissue function. Given the intricate 3D architecture of native tissues, 3D bioprinting aims to build complex geometries that would otherwise be unobtainable through traditional methods, seeking to create viable tissues and organs. Two major hurdles to bioprinting large tissues are 3D printing extracellular matrix (ECM) proteins at high fidelity and generating the microvasculature necessary to maintain cell viability. The mechanical instability of soft (E < 150 kPa) ECM proteins causes significant deformation during printing that hinders the immediate creation of patent microvasculature. Recently, improvements to our 3D bioprinting method Freeform Reversible Embedding of Suspended Hydrogels (FRESH) have allowed us to print ECM components like collagen with a high degree of geometric complexity at a fidelity down to 20 μm.
With a major obstacle largely addressed, we have begun to work towards developing an in vitro vascularization system for vessel templates FRESH printed from collagen. This system is based on leveraging two phenomena in tissue engineering – microporosity and cellular remodeling. By increasing tissue scaffold porosity, we show an increase rate of diffusion through a collagen matrix translates to improved cellular viability while in vivo data shows porosity improves host-driven angiogenesis. By introducing endothelial cells to our porous bioprinted vessel templates, we seek to create an in vitro vascularization system that results in a large, vascularized tissue for transplantation.
Dynamic Loading of Human Engineered Heart Tissue Enhances Contractile Function and Drives Desmosome-linked Disease Phenotype
Jaci Billey, MS
Carnegie Mellon University, Regenerative Biomaterials Therapeutic Group (Adam Feinberg PhD), PhD Student
Abstract: Heart failure is a significant concern affecting more than 5 million adults in the U.S. alone. Hemodynamic loads, including preload (stretch on heart muscle) and afterload (pressure the heart must work against to eject blood), can lead to maladaptive structural and functional changes in the heart, specifically ventricular dilation and reduced force generation. Engineered heart tissues (EHTs) have the potential to provide insight into loading-induced disease progression but current 3D EHT approaches are constrained (length is held constant), which limits their ability to model the altered loads experienced during heart failure, the tissue morphological changes that occur, and the consequences that these have on heart muscle tissue force generation. Here, we have developed a dynamic EHT (dyn-EHT) model that addresses these limitations by integrating EHTs with an elastic polydimethylsiloxane (PDMS) strip, which provides active preload and a contractile force measurement based on strip bending during EHT contraction. Our results demonstrate that dynamic loading is beneficial in wild-type EHTs leading to improved alignment, conduction velocity, and contractility. For disease modeling, we use hiPSC–derived cardiomyocytes from a patient with arrhythmogenic cardiomyopathy due to mutations in desmoplakin. We demonstrate that manifestation of this desmosome-linked disease state requires the dyn-EHT conditioning and that it cannot be induced using 2D or standard 3D EHT approaches. Thus, a dynamic loading strategy is necessary to provoke a disease phenotype (tissue lengthening, reduction of desmosome counts, and reduced contractility), which are akin to primary endpoints of clinical disease, such as chamber thinning and reduced cardiac output.
Mathematical and Computation Models
Interpretable machine learning for biological discovery
Susan Liao, PhD
NYU Courant Institute of Mathematical Sciences, Postdoctoral Fellow with Jef Boeke, PhD, and Additional Ventures LSRF Fellow
Abstract: Neural networks hold great promise for deciphering complex biological logic due to their expressive nature that can describe a breadth of relationships in data that can be quantitatively described through mathematical functions. However, despite great success with predicting outcomes, most neural networks fail to advance biological discovery as they were designed with primary goal of prediction accuracy. Despite excellent predictions, the vast majority of neural networks cannot explain how they arrived these predictions. It is difficult to trust or verify whether these predictions reflect true biological relationships or instead capture biases in the dataset. Uninterpretable neural networks thus provide limited mechanistic insight into underlying biological processes. Furthermore, predictions from an uninterpretable network may not generalize, failing to predict outcomes from data that it has not seen before, limiting the utility of the network in basic research or clinical settings. There is a clear need to design interpretable neural networks that will enable biologists to generate and test novel hypotheses from their data. In this presentation I will present our progress towards this goal, focusing on how advances in data acquisition and data analysis can improve neural network interpretability. These innovations will address interpretability concerns across a wide range of biological questions involving gene expression in both development and disease.
Roles of negative and positive feedback in cardiovascular adaptations
Linda Irons, PhD
Department of Biomedical Engineering, Yale University, Postdoctoral fellow with Jay Humphrey, PhD
Abstract: Single ventricle defects result in altered hemodynamics and reduced blood oxygenation, both of which can stimulate remodeling responses throughout the systemic and pulmonary circulations. Such changes, in turn, can adversely affect the delivery of oxygenated blood within end organs at appropriate pressures and flows, hence contributing to progressive morbidity and mortality. There is, therefore, a pressing need to understand not just how the hemodynamics differs in single ventricle disease, but also how these alterations drive widespread remodeling that is often maladaptive. Toward this end, we have developed computational models “from transcript to tissue” to simulate how cell-perceived stimuli result in particular transcriptional changes that in turn drive tissue level changes in structure and function. Whereas homeostatic processes drive adaptive remodeling through negative feedback, compromised or lost hemostasis results in maladaptive responses characterized by positive feedback.
Stem Cell Models and Applications
Roadmap to efficiently generate human artery and vein endothelial cells from pluripotent stem cells
Kyle Loh, PhD
Stanford University, Assistant Professor and The Anthony DiGenova Endowed Faculty Scholar
Abstract: Endothelial cells—encompassing molecularly-distinct arteries versus veins—pervade all tissues and have manifold roles in health and disease, and also likely contribute to congenital heart defects. What instigates human endothelial cell diversity and how can we recreate such diversity in vitro? Here we efficiently differentiate human pluripotent stem cells through a sequence of branching lineage choices into artery or vein endothelial cells, within 3-4 days. This roadmap encompasses the stepwise changes in extracellular signals, gene expression, chromatin state and cell-surface markers during specialization of artery vs. vein cells from their mesodermal precursors, including a two-step process for vein specification. This thus provides an informative reference map for human artery and vein development. The newfound capability to rapidly and efficiently generate human artery-specific and vein-specific endothelial cells en masse should avail tissue engineering, disease modeling and other diverse applications that hinge on a large-scale supply of human endothelial cells.
SARS-CoV-2 infection of human iPSC-derived cardiac cells predicts novel cytopathic features in hearts of COVID-19 patients
Serah Kang, PhD
UCSF & Gladstone Institutes, Postdoctoral Fellow (McDevitt Lab)
Abstract: Although COVID-19 causes cardiac dysfunction in up to 25% of patients, its pathogenesis remains unclear. Exposure of human iPSC-derived heart cells to SARS-CoV-2 revealed productive infection and robust transcriptomic and morphological signatures of damage, particularly in cardiomyocytes. Transcriptomic disruption of structural proteins corroborated adverse morphologic features, which included a distinct pattern of myofibrillar fragmentation and numerous iPSC-cardiomyocytes lacking nuclear DNA. Human autopsy specimens from COVID-19 patients displayed similar sarcomeric disruption, as well as cardiomyocytes without DNA staining. These striking cytopathic features provide new insights into SARS-CoV-2 induced cardiac damage, offer a platform for discovery of potential therapeutics, and raise serious concerns about the long-term consequences of COVID-19.
Genetics and Regulatory Elements
Predicting the disruption of 3D genome folding in congenital heart defects
Maureen Pittman, BS, UCSF & Gladstone Institutes, PhD Student with Katie Pollard, PhD
Abstract: Many disease-associated genetic variants are located in putative regulatory elements, suggesting a major role for transcriptional misregulation in disease. This is especially relevant to developmental disorders, in which the disruption of finely-tuned transcriptional networks is likely to have acute consequences to cell differentiation and morphogenesis. The fidelity of gene regulation is enforced partly by the three-dimensional organization of the genome, which is arranged such that regulatory elements and their target promoters are able to make contact in the appropriate contexts. Structural variants have the potential to disrupt that system by changing the contact frequency of promoters to their regulatory elements. Here, we hypothesize that such variants may contribute to the formation of congenital heart defects. Using structural variants identified by the Pediatric Cardiac Genomics Consortium, we use a convolutional neural network to predict the resulting change in chromatin contact frequency and prioritize variants for experimental validation.
Genome-wide maps of enhancer regulation connect risk variants to disease genes
Jesse Engreitz, PhD, Stanford University, Assistant Professor
Abstract: Enhancers harbor thousands of variants associated with common diseases and traits. Each of these variants could reveal insights into disease mechanisms or therapeutic targets. Yet, it has proven difficult to connect these variants to their molecular functions because we have lacked tools to systematically map which enhancers regulate which genes in which cell types. Here we use new CRISPR methods to perturb >4,500 enhancer-gene connections in several cell types. We show that an Activity-by-Contact (ABC) Model of enhancer function — involving multiplying enhancer activity by enhancer-promoter contact — can accurately predict these experimental perturbations based on easily obtained maps of chromatin state. We apply this ABC Model to create enhancer-gene maps in 131 cell types and tissues, and use these maps to interpret the functions of genetic variants associated with common diseases and complex traits. We find that over half of causal noncoding GWAS variants likely act via effects on enhancers. Variants associated with cardiovascular diseases connect to genes in endothelial cells and cardiomyocytes. These ABC maps provide a generalizable strategy to connect common disease risk variants in enhancers to target genes, and will help to understand the genetic etiology of congenital heart defects.
We're sorry, there are no results for your query. Please try again.